topic 1.3: Energy and equilibria
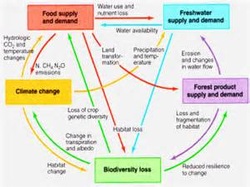
Ecosystems are dynamic interrelated collections of living and non-living components organized in self-regulating units. An ecosystem is a unit because it has boundaries and can be distinguished from its surroundings. The living and non-living components affect each other in complex exchanges of energy, nutrients and wastes. It is these dynamic exchanges, both fast and slow, which provide ecosystems with their distinct identities. Because of these distinct features ecosystems themselves represent part of the earth’s biodiversity. The characteristic exchanges within an ecosystem are called ecosystem functions and in addition to energy and nutrient exchanges, involve decomposition and production of biomass. The complex interdependencies which develop within or among ecosystems often create emergent properties, or characteristics that cannot be predicted from the component parts alone.
Open systems tend to exist in a state of balance or quilibrium. Equilibrium is important for a system as it avoids sudden changes in a system. However, this does not mean that all systems are none changing. If change exists it tends to exist between certain peramiters. Equilibrium states in two ways state and steady.
Static Equilibrium is a system in a steady state because the inputs and outputs that affect it approximately balance over a long period of time.
The laws of thermodynamics govern the flow of energy in a system. This flow of energy gives the system the ability to do work. Systems can exist in alternative stable states or as equilibria between which there are tipping points.
Destabilizing positive feedback mechanisms will drive systems toward these tipping points, whereas stabilizing negative feedback mechanisms will resist such changes.
This unit will take a minimum of 3 hours.
Significant Ideas
- The laws of thermodynamics govern the flow of energy in a system and the ability to do work
- Systems can exist in alternative stable states or as equilibria between which there are tipping points
- Destabilizing position feedback mechanisms will drive systems toward these tipping points, whereas stabilizing negative feedback mechanisms will resist such changes
Big questions:
- What strengths and weaknesses of the systems approach and the use of models have been revealed through this topic
- How are the issues addressed in this topic of relevance to sustainability or sustainable development?
- The principle of conservation of energy can be modeled by the energy transformations along food chains and energy production systems: what are the strengths and limitations for such models?
- How do the delays involved in feedback loops make it difficult to predict tipping points and add to the complexity of modelling systems?
- Do the benefits of the models used to predict tipping points outweigh their limitations?
- How does sustainability reduce the change that tipping points will be reached?
Knowledge and Understanding
1.3.U1 The first law of thermodynamics is the principle of conservation of energy, which states that energy in an isolated system can be transformed but cannot be created or destroyed
[The use of examples in this sub-topic is particularly important so that the abstract concepts have a context in which to be understood]
[The use of examples in this sub-topic is particularly important so that the abstract concepts have a context in which to be understood]
- Define the first law of thermodynamics
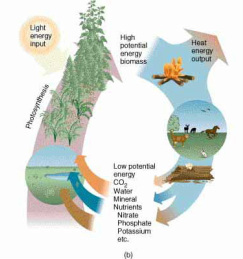
1st: energy is neither created nor destroyed, only changes forms. This is the principle of conservation of energy, Energy in an isolated system can be transformed but cannot be created or destroyed.
(Both laws should be examined in relation to the energy transformations and maintenance of order in living systems.)
- Ex: solar radiation -> sugars -> chemical energy -> chemical energy again
(Both laws should be examined in relation to the energy transformations and maintenance of order in living systems.)
1.3.U2 The principle of conservation of energy can be modelled by the energy transformations along food chains and energy production systems.
- Explain how the first law of thermodynamics apply to a food chain and an energy production system. Use a systems diagram to aid in your explanation.
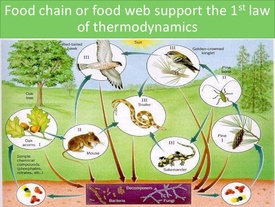
The principle of conservation of energy states that energy cannot be created or destroyed, i.e. in an isolated system, the total energy before transformation is equal to the total energy transformation. Energy can only be changed from one form to another. Energy for the functioning of an ecosystem comes from the Sun. Solar energy is absorbed by plants where in it is converted to stored chemical energy.
1.3.U3 The second law of thermodynamics states that the entropy of a system increases over time. Entropy is a measure of the amount of disorder in a system. An increase in entropy arising from energy transformations reduces the energy available to do work.
- Define the second law of thermodynamics
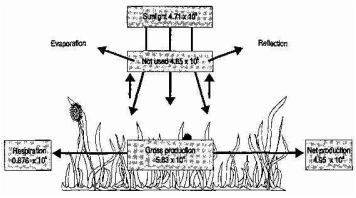
The second law of thermodynamics states that whenever energy is transformed, there is a loss energy through the release of heat.
Entropy of a system increases overtime. Entropy is a measure of the amount of disorder in a system. An increase in entropy arising from energy transformations reduces the energy available to do work.
Entropy of a system increases overtime. Entropy is a measure of the amount of disorder in a system. An increase in entropy arising from energy transformations reduces the energy available to do work.
- Living systems are only maintained through constant input of new energy from the Sun
- Entropy is simply a quantitative measure of what the second law of thermodynamics describes: the dispersal of energy in a process in our material world
1.3.U4 The second law of thermodynamics explains the inefficiency and decrease in available energy along a food chain and energy generation systems.
- Explain how the second law of thermodynamics apply to a food chain and an energy production system. Use a systems diagram to aid in your explanation.
- Outline the concept of entropy and explain how it reduces the energy available to do work in the systems.
- Distinguish between the first and second law of thermodynamics.
- Distinguish between how the first and second laws of thermodynamics impact food chains.
This occurs when energy is transferred between trophic levels as illustrated in a food chain When one animal feeds off another, there is a loss of heat (energy) in the process. Additional loss of energy occurs during respiration and movement. Hence, more and more energy is lost as one moves up through trophic levels. This fact lends more credence to the advantages of a vegetarian diet. For example, 1350 kilograms of corn and soybeans is capable of supporting one person if converted to beef. However, 1350 kilograms of soybeans and corn utilized directly without converting to beef will support 22 people!
|
1.3.U5 As an open system, an ecosystem will normally exist in a stable equilibrium, either in a steady-state equilibrium or in one developing over time (for example, succession), and maintained by stabilizing negative feedback loops.
[A stable equilbrium is the condition of a system in which there is a tendency for it to return to the previous equilibrium following disturbance]
[A steady-state equilibrium is the condition of an open system in which there are not changes over the longer term, but in which there may be oscillations in the very short term]
[A stable equilbrium is the condition of a system in which there is a tendency for it to return to the previous equilibrium following disturbance]
[A steady-state equilibrium is the condition of an open system in which there are not changes over the longer term, but in which there may be oscillations in the very short term]
- Explain the stable and steady-state equilibrium
- Using examples, explain how complexity of a system can lead to its stability.
- Explain the nature of equilibrium
- Describe what is meant by steady-state equilibrium and explain how it is stabilized by negative feedback.
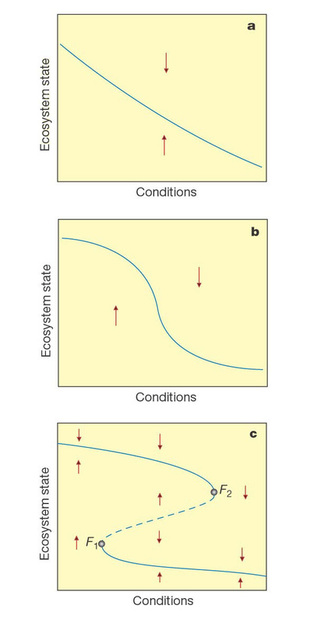
As an open system, an ecosystem will normally exist in a stable equilibrium, either in a steady state equilibrium or in one developing over time (for example Succession), and maintain y stabilizing negative feedback loops.
- Steady-state: in open systems, continuous inputs and outputs of energy and matter, system as a whole remains in a constant state, no long term changes. There may be oscillations in the very short term.
- Static: no change over time; when the state of equilibrium is distributed, the system adapts a new equilibrium; can’t occur in living systems
- Stable: the system returns to the same equilibrium after disturbances
- Unstable: system returns to a new equilibrium after disturbances
1.3.U6 Negative feedback loops (stabilizing) occur when the output of a process inhibits or reverses the operation of the same process in such a way as to reduce change—it counteracts deviation.
- Describe and draw systems diagrams of two examples of negative feedback
- Discuss how negative feedback can drive a system towards the tipping point
- Explain how negative feedback loops stabilize an ecosystem to produce steady state equilibrium.
- Discuss how negative feedback loops maintain equilibrium

Ecosystem feedback is the effect that change in one part of an ecosystem has on another and how this effect then feeds back to effect the source of the change inducing more or less of it. These feedback loops form the basic dynamics for regulating the state of the ecosystem.
In order for a system to maintain a steady state or average condition the system must possess the capacity for self-regulation. Self-regulation in many systems is controlled by negative feedback and positive feedback mechanisms.
Negative-feedback mechanisms control the state of the system by dampening or reducing the size of the system's elements or attributes. Positive-feedback mechanisms feed or increase the size of one or more of the system's elements or attributes over time.
Negative (stabilizing): tends to neutralize or counteract any deviation from an equilibrium and tends to stabilize systems. The systems gets better or goes back to normal..
Resistance to drastic changes and thus stability in natural ecosystems are maintained in part by negative feedback systems. These are familiar to you through considering the system that regulates the heat in your home. When the temperature in your house decreases below some set point, the thermostat that senses temperature is activated to send a "turn on" message to your furnace. Then, once the temperature rises above another set point, the thermostat sends a message to the furnace, telling it to shut off. Thus, the temperature in your house is maintained within bounds. This illustrates a negative feedback system. Negative feedback systems act to maintain homeostasis within systems; that is, to keep them in a reasonably constant state.
In order for a system to maintain a steady state or average condition the system must possess the capacity for self-regulation. Self-regulation in many systems is controlled by negative feedback and positive feedback mechanisms.
Negative-feedback mechanisms control the state of the system by dampening or reducing the size of the system's elements or attributes. Positive-feedback mechanisms feed or increase the size of one or more of the system's elements or attributes over time.
Negative (stabilizing): tends to neutralize or counteract any deviation from an equilibrium and tends to stabilize systems. The systems gets better or goes back to normal..
Resistance to drastic changes and thus stability in natural ecosystems are maintained in part by negative feedback systems. These are familiar to you through considering the system that regulates the heat in your home. When the temperature in your house decreases below some set point, the thermostat that senses temperature is activated to send a "turn on" message to your furnace. Then, once the temperature rises above another set point, the thermostat sends a message to the furnace, telling it to shut off. Thus, the temperature in your house is maintained within bounds. This illustrates a negative feedback system. Negative feedback systems act to maintain homeostasis within systems; that is, to keep them in a reasonably constant state.
1.3.U7 Positive feedback loops (destabilizing) will tend to amplify changes and drive the system towards a tipping point where a new equilibrium is adopted.
- Compare and contrast positive and negative feedback loops.
- Describe and draw systems diagrams of two examples of positive feedback.
- Explain tipping point
- Describe what often characterizes a tipping point.

Positive (destabilizing): results in a further decrease of output and the system is destabilized and pushed into a new state of equilibrium. The situation gets worse.
Positive feedback stimulates change and it is responsible for the sudden appearance of rapid changes within ecosystems. When part of the system increases, another part of the system also changes in a way that makes the first part increase even more. Positive feedback is a source of instability and change as it can drive the system outside of its equilibrium. As an example more population leads to more births, and more births lead to an increasing population creating a compounding effect over time.
The self-regulation of natural systems is achieved by the attainment of equilibrium through feedback systems.
Feedback links involve time lags.
Positive feedback stimulates change and it is responsible for the sudden appearance of rapid changes within ecosystems. When part of the system increases, another part of the system also changes in a way that makes the first part increase even more. Positive feedback is a source of instability and change as it can drive the system outside of its equilibrium. As an example more population leads to more births, and more births lead to an increasing population creating a compounding effect over time.
The self-regulation of natural systems is achieved by the attainment of equilibrium through feedback systems.
Feedback links involve time lags.
1.3.U8 The resilience of a system, ecological or social, refers to its tendency to avoid such tipping points and maintain stability
[Emphasis should be placed on the relationships between resilience, stability, equilibria and diversity]
[Emphasis should be placed on the relationships between resilience, stability, equilibria and diversity]
- Explain what is meant by resilience of a system
- Explain how resilience avoids tipping points.
- Discuss natural and human factors that affect resilience in systems.
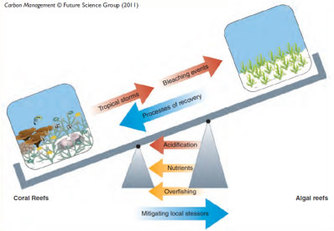
Resilience is the ability of a system to return to its initial state after a disturbance
High resilience = return to equilibrium
Low resilience = enter a new state/equilibrium
Resilience is usually good but can be bad
A tipping point is a critical threshold when even a small change can have dramatic effects and cause a disproportionately large response in the overall system. Positive feedback loops are destabilizing and tend to amplify changes and drive the system towards a tipping point where a new equilibrium is adopted. Most projected tipping points are linked to climate change and represent points beyond which irreversible change or damage occurs. Increases in CO2 levels that would lead to increased global mean temperature, causing melting of the ice sheets and permafrost. Reaching such a tipping would, for example, cause long-term damage to societies, the melting of Himalayan mountain glaciers, and a lack of freshwater in many Asian societies.
Positive feedback loops (destabilizing) will tend to amplify changes and drive the system towards a tipping point where a new equilibrium is adopted. A tipping point is the minimum amount of change within a system that will destabilize
it, causing it to reach a new equilibrium or stable state.
Systems at threat from tipping points include:
High resilience = return to equilibrium
Low resilience = enter a new state/equilibrium
Resilience is usually good but can be bad
A tipping point is a critical threshold when even a small change can have dramatic effects and cause a disproportionately large response in the overall system. Positive feedback loops are destabilizing and tend to amplify changes and drive the system towards a tipping point where a new equilibrium is adopted. Most projected tipping points are linked to climate change and represent points beyond which irreversible change or damage occurs. Increases in CO2 levels that would lead to increased global mean temperature, causing melting of the ice sheets and permafrost. Reaching such a tipping would, for example, cause long-term damage to societies, the melting of Himalayan mountain glaciers, and a lack of freshwater in many Asian societies.
Positive feedback loops (destabilizing) will tend to amplify changes and drive the system towards a tipping point where a new equilibrium is adopted. A tipping point is the minimum amount of change within a system that will destabilize
it, causing it to reach a new equilibrium or stable state.
Systems at threat from tipping points include:
|
|
1.3.U9 Diversity and the size of storages within systems can contribute to their resilience and affect their speed of response to change (time lags).
- Discuss what factors contribute to resilience and how humans affect these factor
- Explain how lag time can add complexity to modeling and predicting tipping points
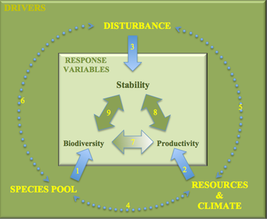
Resilience of a system will depend on its structure.
- The more diverse/complex an ecosystem, the more resilient it tends to be (more interactions between species). The greater the species biodiversity of an ecosystem, the greater the likelihood there is a species that can replace another if it dies (to maintain equilibrium).
- The greater the genetic diversity within a species, the greater resilience. A monoculture of wheat or rice can be wiped out by disease if none of the plants have genetic resistance.
- Species that can shift geographic ranges are more resilient.
- The climate affects resilience. In the Arctic, regeneration/growth of plants is slow (low temps slow down photosynthesis/cell respiration). In tropical rain forests, growth rates are fast (light, temp, water are not limiting factors).
- The faster the rate at which a species can reproduce means recovery is faster. r-strategists (fast reproductive rate) can recolonize the system better than K-strategists (slow reproducers).
1.3.U10 Humans can affect the resilience of systems through reducing these storages and diversity
[Examples of human impacts and possible tipping points should be explored].
[Examples of human impacts and possible tipping points should be explored].
- Explain how humans can add complexity to the resilience of a system
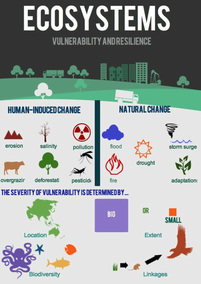
Humans can remove or mitigate threats to the system (pollution, invasive species) – resulting in faster recovery.
Humans can reduce size of storages by harvesting wood, fish and other natural resuources. Humans can also reduce diversity by species extinction which leads to less resilience.
Humans can reduce size of storages by harvesting wood, fish and other natural resuources. Humans can also reduce diversity by species extinction which leads to less resilience.
1.3.U11 The delays involved in feedback loops make it difficult to predict tipping points and add to the complexity of modelling systems.
[A tipping point is the minimum amount of change within a system that will destabilize it, causing it to reach a new equilibrium or stable state]
[A tipping point is the minimum amount of change within a system that will destabilize it, causing it to reach a new equilibrium or stable state]
- Explain how delays in feedback loops can add complexity to modeling and predicting tipping points

Within limits an ecosystem can recover and re-establish its equilibrium. When the disturbance is too great the ecosystem reaches a tipping point. The critical point in a situation, process, or system behold which a significant and often unstoppable effect or change takes place. Past the tipping point the ecosystem can't re-establish its equilibrium.
Positive feedback pushes the ecosystem to a new equilibrium where there are significant changes to biodiversity and services it provides
Positive feedback pushes the ecosystem to a new equilibrium where there are significant changes to biodiversity and services it provides
Applications
1.3.A1 Explain the implications of the laws of thermodynamics to ecological systems.
Two thermodynamic laws govern the flow of energy within ecological systems. The first law states that energy can be transformed from one form to another (e.g., conversion of solar energy to chemical energy by photosynthesis), but cannot be created or destroyed. The second law establishes that energy transformation processes are not 100% efficient. These laws dictate that a large proportion of the chemical energy, approximately 90%, transferred between feeding (trophic) levels within a system is converted to heat energy which is of limited value to the biotic portion of the system. The energy "loss" between feeding levels results from an inefficient transfer of organic matter (e.g., gaseous, urinary and fecal losses) and the energy required for internal maintenance of organisms (i.e., maintenance energy). For example, only a portion of the solar energy converted into chemical energy by photosynthesis is realized as growth because a portion is utilized in respiration. Similarly, animals utilize a large portion of the total energy ingested for basal metabolism thereby diminishing the amount of energy available for growth or transfer to subsequent feeding levels within the system
1.3.A2 Discuss the resileance in a variety of systems
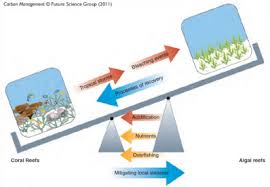
Resilience is the capacity of an ecosystem to respond to a disturbance. Resilience is usually defined as the capacity of an ecosystem to absorb disturbance without shifting to an alternative state and losing function and services. Disturbances can include fires, flooding, windstorms, insect population explosions, and human activities such as deforestation, fracking of the ground for oil extraction, pesticide sprayed in soil, and the introduction of exotic plant or animal species.
Some disturbances can significantly affect an ecosystem and can cause an ecosystem to reach a threshold beyond which some species can not recover. Human activities that adversely affect ecosystem resilience such as reduction of biodiversity, exploitation of natural resources, pollution, land-use, and anthropogenic climate change are increasingly causing changes in ecosystems, often to less desirable conditions.
Diversity and the size of storage's withing systems can contribute to their resilience and affect their speed of response to change (time lag)
You need discuss resilience in a variety of systems
Some disturbances can significantly affect an ecosystem and can cause an ecosystem to reach a threshold beyond which some species can not recover. Human activities that adversely affect ecosystem resilience such as reduction of biodiversity, exploitation of natural resources, pollution, land-use, and anthropogenic climate change are increasingly causing changes in ecosystems, often to less desirable conditions.
Diversity and the size of storage's withing systems can contribute to their resilience and affect their speed of response to change (time lag)
You need discuss resilience in a variety of systems
1.3.A3 Evaluate the possible consequences of tipping points
Evaluate: Make an appraisal by weighing up the strengths and limitations.
Evaluate: Make an appraisal by weighing up the strengths and limitations.
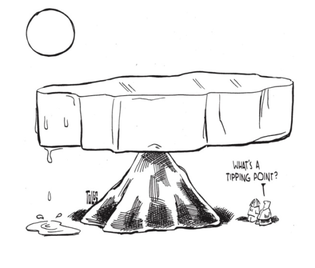
The resilience of a system, ecological or social, refers to its tendency to avoid such tipping points and maintains stability. A tipping point is the minimum amount of change within a system that will destabilize it, causing it to reach a new equilibrium or stable state.
An environmental tipping point is a part of the human-environment system that can lever far-reaching change in the system. A change at the tipping point sets in motion mutually reinforcing feedback loops that propel the system on a completely new course.
The delays involved in feedback loops make it difficult to predict tipping points and add to the complexity of modelling systems
You need to understand the relationships between resilience, stability, equilibria and diversity using specific examples to illustrate interactions.
An environmental tipping point is a part of the human-environment system that can lever far-reaching change in the system. A change at the tipping point sets in motion mutually reinforcing feedback loops that propel the system on a completely new course.
The delays involved in feedback loops make it difficult to predict tipping points and add to the complexity of modelling systems
- Lake Eutrophication:
- Extinction of a Keystone Species:
- Removal of elephants from a savannah ecosystem can result in irreversible damage to that system.
- Coral Reef Death: If ocean acidity levels increase, the reef coral dies and cannot regenerate.
You need to understand the relationships between resilience, stability, equilibria and diversity using specific examples to illustrate interactions.
Key Terms
positive feedback
tipping-point resilient stability diversity Laws of Thermodynamics Conservation of Energy |
negative feedback
entropy destabilizing stabilizing work global warming albedo keystone species |
sustainability
thermodynamics energy transfer equilibria transformation transfer predator/prey monoculture |
ecosystem
equilibrium storage energy static equilibrium unstable equilibria transformations homeostasis |
genergy efficiency
flows steady-state equilibrium energy transformation stable equilibria complexity precautionary principle eutrophication |
Classroom Resources
Energy Flow Diagram homework
Equilibrium and Feedback Loops handout
Is Earth Nearing an Environmental Tipping Point - Scientific America
Tipping Point Case Studies
Thermodynamics of Food Investigation
Negative or Positive Feedback Activity
Predator - Prey Simulation in class activity
Case Studies
Antarctic krill
Tall grass prairies in the US
Energy Flow Diagram homework
Equilibrium and Feedback Loops handout
Is Earth Nearing an Environmental Tipping Point - Scientific America
Tipping Point Case Studies
Thermodynamics of Food Investigation
Negative or Positive Feedback Activity
Predator - Prey Simulation in class activity
Case Studies
Antarctic krill
Tall grass prairies in the US
Powerpoint and Notes Adapted from Brad Kremer, P Brooks and Ms. McCrindle
Your browser does not support viewing this document. Click here to download the document.
Your browser does not support viewing this document. Click here to download the document.
Correct use of terminology is a key skill in ESS. It is essential to use key terms correctly when communicating your understanding, particularly in assessments. Use the quizlet flashcards or other tools such as learn, scatter, space race, speller and test to help you master the vocabulary.
Useful links
Nitche Science 1.3: Energy and equilibria- Thermodynamics
Nitche Science 1.3: Energy and equilibria- Resilience and Tipping Point
Feedback Loops - Starting Point
Sea Ice & Thermodynamics - National Snow and Ice Center
National Ice and Snow Databank - National Snow and Ice Center
What is Albedo? - The Encyclopedia of Earth
The Globe Program
EcoTipping Point Project
Entertaining Thermodynamics Song
Laws of Thermodynamics Explained
What is Entropy?
Positive and Negative Feedback
Wkst: Feedback Mechanisms
Identifying Feedback Practice
In The News
Hurricane Sandy: Global warming, pure and simple - SALON 31 Oct 2012
Energy Economics in Ecosystems - The Knowledge Project
International-Mindedness
- The us of models facilitates international collaboration in science by removing language barriers that may exits.
TOK:
- The laws of thermodynamics are examples of scientific laws - in which ways do scientific laws differ from the laws of human science subjects, such as economics?
Video Clips
Biological processes depend on energy flow through the Earth system.
This video is about how we don't just need energy to power our lives, we need *low entropy* energy! This is also known as "free energy", and one way of thinking about entropy is as the lack of free energy. The sun provides us with useful, low entropy, energy in the form of photons of visible light, which are then absorbed and converted into plants, sugar, complex molecules, etc, before being radiated back into space as heat, which has much higher entropy.
This video explains the 1st and 2nd laws of thermodynamics as they relate to energy exchanges with environmental systems.
In this episode of Crash Course Chemistry, Hank goes over the ideas of keeping your life balance... well, your chemical life. Equilibrium is all about balance and today Hank discusses Chemical Equilibrium, Concentration, Temperature, and Pressure. Also, he'll chat about Le Chatalier's Principle and Fritz Habe
While feedback loops are a bummer at band practice, they are essential in nature. What does nature’s feedback look like, and how does it build the resilience of our world? Anje-Margriet Neutel describes some common positive and negative feedback loops, examining how an ecosystem’s many loops come together to make its ‘trademark sound
Watch the following video to learn about: -Positive feedback loops -Negative feedback loops -How competing feedback loops may influence climate change
Paul Andersen explains how ecosystems respond to disruptions. Disruptions can cause changes in the number and variety of organisms. It can also lead to migration, extinction or even speciation.
"A short animated film about the feedback loops likely to lead to catastrophic climate change, by Leo Murray."
From time to time, ecosystems are hit by storms, fires or other types of disturbance. Resilience is the ability of an ecosystem to resist or recover from these events. Resilience preserves ecosystem diversity, productivity and sustainability. Disturbance has more impact on ecosystems that have lost diversity.
For 10,000 years, our world seemed endless. The sky was the limit. But today's world looks much smaller. We've cleared, consumed and polluted our way across the globe. The planet is shrinking. Have we pushed Earth past the tipping point? That's a critical issue the University of Minnesota's Institute on the Environment explores in our second Big Question video. This three-minute multimedia feature draws on research from the article Planetary Boundaries: A Safe Operating Space for Humanity, published this past fall in the journal Nature, and discussed in the current Scientific America
Earth Could Reach Devastating Ecological Tipping Point by 2025
This segment deals with the main positive feedback loops in action in Gaia's complex ecosystems: melting of the ice caps, deforestation, melting of permafrost regions of the planet and the destruction of Ehux: a vital marine algae that is a key component of the earth's climate regulation system.
The scientific evidence appears (overwhelmingly) to indicate that earth (Gaia) may be inexorably approaching or perhaps PAST the tipping point and on it's way to a new climate regime not seen on earth for 55 million years. It will be a climate that is, on average, much hotter, more chaotic and violent than what we have experienced for the entire tenure of our species.
The scientific evidence appears (overwhelmingly) to indicate that earth (Gaia) may be inexorably approaching or perhaps PAST the tipping point and on it's way to a new climate regime not seen on earth for 55 million years. It will be a climate that is, on average, much hotter, more chaotic and violent than what we have experienced for the entire tenure of our species.
Rain Forest Tipping Point
In a remote fishing village in the Philippine archipelago, coastal fishers responded to falling fish stocks by working harder to catch them. The combination of dynamite, longer workdays, and more advanced gear caused stocks to fall faster. On the edge of crisis, this small community decided to create a no-take marine sanctuary on 10% of its coral-reef fishing grounds. This initiative sparked a renaissance of not only their fishery, but also their cherished way of life
Plenty of people have an opinion on whether the precautionary principle is good or bad, but what exactly is it?